
Nested below the ground, at the border between Switzerland and France, a giant awaits. Its 27-kilometer long body is capable of shooting two of the fastest moving beams on Earth. The secrets of the Universe and the most energetic collision since the beginning of time will happen right in the four eyes of the giant when the beams meet. Right now it is licking its wounds, inflicted due to an electrical fault, but it will wait a few more months when its roar will be heard, and the mysteries of the Universe will be unravelled. It is the Large Hadron Collider.

Hadrons are subatomic particles that subject to the strong force. One example of such heavy hadrons are lead ions (with atomic number 82), stripped of all its electrons. This very LHC is capable of smashing such large hadrons with each other, hence the name. This baby can pack up to 14 TeV into proton-proton collision, and 1150 TeV into lead-lead collision. And its four “eyes” are called A Large Ion Collider Experiment (ALICE), Large Hadron Collider beauty (LHCb), Compact Muon Solenoid (CMS), and A Toroidal LHC ApparatuS (ATLAS).

Probably the biggest structure humans have build in the last thousand years, this baby cost so much that international collaboration is needed. It is so popular that the YouTube video of the LHC rap has reached over 5 million viewers. The questions in physics that might be answered by it are so big that over 10 thousand scientists and engineers are working on it. Indeed the only reason that the behemoth is built is to find out if there is a Higgs boson, if there more fundamental particles than quarks and leptons, if nature is supersymmetric, what dark matter is and, finally, why matter and antimatter do not exist in the same amount.
Let’s look at these questions one by one and see why they are considered important, starting with the Higgs boson. To understand the Higgs, we must understand the Standard Model which is currently the most accurate physics theory that has successfully predicted all of the subatomic particles found in previous accelerators. In particular, the story of the W and Z bosons are of interesting consequences.

In the standard model, there are four fundamental forces with three of them united with an accurate quantum mechanical description. Among them, the electromagnetic force and the weak nuclear force are the first ones to be unified into the electroweak theory. In this theory, the carriers for the weak nuclear force, two W-bosons and Z-boson, are predicted and found a few years later! However in the process of finding the theory, physicists assumed that the carriers of the weak force are massless bosons, and deduced from the theory 4 massless bosons, 3 for the weak force and the photon. To account for the mass of the W and Z bosons, Peter Higgs proposed a scalar field, called the Higgs field, that is everywhere and interacts with particles to give them rest mass. Just like alpinekat rapped,
“Some particle slows down and others race, straight through like the photon, it has no mass, but something heavy like the top quarks, it’s dragging its---”
It is kind of like adding air resistance to projectile motion. As with every field, quantum mechanics implies that a particle is associated with it. The Higgs boson becomes central to the Standard Model for being able to neatly explain the origin of mass. It is for this reason that the presence of Higgs bosons is a very popular theory. Being the only particle in the Standard Model that has not yet been observed only adds excitment to the mystery. It is now that the LHC will see if the elusive and heavy Higgs boson actually exists. The interesting possibility is that if the LHC does not find the Higgs, numerous other less popular theories would then be able to pit their predictions fitting what the LHC does find.

One such other theory is the preon theory, that quarks and leptons are composed of smaller, more fundamental particles called preons. Currently, the size of quarks and electrons in the Standard Model are regarded as zero. Experiments however, only shows that they are smaller than 10-18 metres. Now the LHC enables us to see at smaller scales, down to 2*10-19 metres, 10000 smaller than a proton. It may finally reveal that quarks or electrons have a finite size and be made of smaller stuffs, like preons. It is also tempting to think that there can be a simple explanation for the three families of quarks and leptons observed, as history had shown that the periodic table of elements hints of electron configuration. History has also shown that radioactivity and atoms changing from one to another hints at a nuclear structure with protons and neutrons, not unsimilar to the decay of heavy elementary particle like top quarks and tau into their lighter counterparts. Whatever the hints and possibilities, without an experimental observation of preons, all we can do is to wait and see.
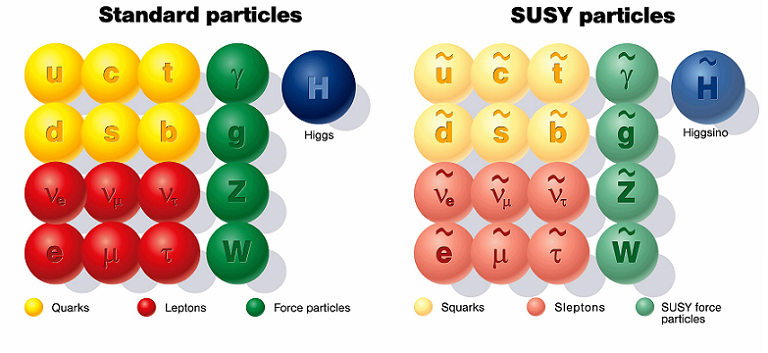
Now that we know the main reason the LHC is build for, it is time for the giant to shed light on what we think is possible. SUperSYmmetry (SUSY) is a principle that states for every fermion (particles with spin n/2 and obey the Pauli Exclusion Principle like quarks and leptons) there is a corresponding boson (particles with integer spin and can occupy the same space with the same energy like photons) and vice-versa so we have electrons and selectrons, photons and photinos. It is symmetric because when we switch the bosons and fermions, the world is still the same. However, since none of the supersymmetric partners have been found yet, it is clearly not a perfect symmetry in terms of mass like matter-antimatter.
One would say that supersymmetry is absurd; if all the electrons are replaced by selectrons that can pass through each other, imagine what will happen to molecules. However, there are many theories that can make use of supersymmetry to explain various things. Most Grand Unified Theories (GUT) predict that the electromagnetic, weak nuclear force and strong nuclear force converge to almost the same strength at around 1015 GeV (compared to 100GeV of electroweak bonding). However, the three forces do not exactly meet at one point. But with supersymmetry, the three forces cut exactly and there is a possible explanation of why the two unifications (GUT and electroweak) happen at so large an energy gap. More than that, if supersymmetric particles are created in the LHC, they will decay until there is the lightest supersymmetric particle (LSP). Therefore, if supersymmetry is true, there will be many LSPs in the universe waiting to be found. It is also this LSP that might be the mysterious dark matter.

Talking about dark matter, it is not a matter that is magically “Dark” or “Evil”. We call it dark initially because it is matter that does not glow, therefore escaping the observation of telescopes. However there is a double meaning to it, dark also means that we do not know much of anything about it, or, that we have too many ideas of what it might be. Embarrassing? You bet. Why not just do away with the concept altogether then?
Well, the reason we need dark matter is to explain how galaxies seem to be able to hold on to their galactic arms of stars. The stars at the outer arms of galaxies are moving too fast to be able to be gravitationally bounded by the luminous matter in the galaxy. So MAssive Compact Halo Objects (MACHOs) like black holes, burned out stars, asteroids and stray planets are possible candidates of dark matter, but Weakly Interactive Massive Particles (WIMPs) like neutrinos, or LSP does fit the bill of being dark matter too.

Unfortunately, both MACHOs and neutrinos encounter problems to be the dark matter needed to explain the galaxy mystery. Using Gravitational Lensing, astronomers determined that the amount of MACHO seems to be too little to account for the dark matter needed. (Gravitational lensing happens when a MACHO passes through the light path of a star, the star appears to brighten for a while then it dimmed back with certain characteristics.) Besides that, the amount of baryonic particles that make up dark matter is limited. Standard primordial nucleosynthesis pins down the value of baryon-to-photon ratio and through astronomical observation, the amount of dark matter greatly exceeds the amount of baryonic particles in the Universe. Neutrinos, on the other hand, are expected to have non-zero rests mass due to the neutrino oscillation. However they move near the speed of light due to their small rest mass and according to computer simulations, that is far too fast to make up dark matter. All these tell us that we do not know a lot about dark matter and therefore, we need more information from the LHC. If the LSP is found, we would be able to test its properties to determine whether it is the main bulk of dark matter. In particular, the ATLAS and CMS will use different designs and concepts to look out for any supersymmetric particle, Higgs boson, preons, or something else entirely different.

When we question what dark matter is, there is a bigger question unresolved, why should there be any matter at all? This can only mean that there is asymmetry between matter and antimatter, which is still a mystery to physicists. In more formal terms, it is called CP violations, or Charge-Parity violations. A Parity transformation is the flip of a sign in spatial coordinates, and matter is exactly the same as antimatter except that both their charge and the direction of their spin are opposite. That is why when CP-violation occurs, it means that there is asymmetry between matter and antimatter. In 1964, the decay in neutral kaons exhibits a small CP-violation. However, physicists expected a greater asymmetry in mesons involving bottom quarks. More recently in 2004, Belle and BaBar, both electron-positron colliders, discovered that the decay of neutral B mesons, made up of bottom and down quarks, shows a preference for matter 100000 times greater than kaons. The antimatter bottom quarks are observed to be 20% less than matter bottom quarks. Belle and BaBar are limited in their energy scale and confined to studying that particular decay. However, the LHCb will look out for the decays involving the bottom quarks (once called beauty quarks) to source out for more CP-violations in order to deepen our understanding of the reason that we are matter instead of all radiation.

Now we are left with ALICE, which looks at the collisions of lead ions. Lead-lead collisions aims to spread a lot of energy over a large volume compared to proton-proton collision. Having the collision energy of 1150 TeV, it can reach back and recreate the situations just 10-12-10-6 seconds after the big bang (the quarks-gluon plasma). Just like a transition phase from solid to liquid, the quarks-gluon plasma is a zero viscosity fluid that cools down to bound quarks at 10-6 seconds after the big bang. This phase transition is what ALICE will study and help in the cosmological mapping of events after the big bang.
Lord Kelvin once said that there is nothing new to be discovered in physics now, all that remains is more and more precise measurement. However, more and more precise measurements reveals newer physics that no one can predict. And no one can predict what exactly the LHC will find. It can confirm come theories, and discredit others, it can answer some questions and/or raise more, it might even alter the paradigm of physics. Whatever it does, it will change the face of physics as we know it.
End note: The web contains an almost endless information and picture of the LHC; I hope that those interested will spend some time to find out more about the LHC as I think it is embarrassing if physics majors don’t know more than the average public readers out there about the most exciting experiment in physics. This article is written partly for that purpose in mind. The book that I based on most is Don Lincoln’s “The Quantum Frontier” 2009 The Johns Hopkins University Press, Baltimore. Another book that helped is Lord Martin Rees’ “New Perspectives in Astrophysical Cosmology” Second Edition 2000 Cambridge University Press. Both books can be found in the Science Library in NUS. Lastly, I hope you enjoyed reading this.
1 comment:
A couple of useful URLs pertaining to one of the books you referenced:
http://www.thequantumfrontier.com
and
http://www.facebook.com/pages/Don-Lincoln/100958137881
Post a Comment